Neuroligin-3 is a protein that plays a critical role in supporting synaptic communication between neurons. These connections enable the formation of thoughts, memories, and emotions, ultimately shaping our experiences.
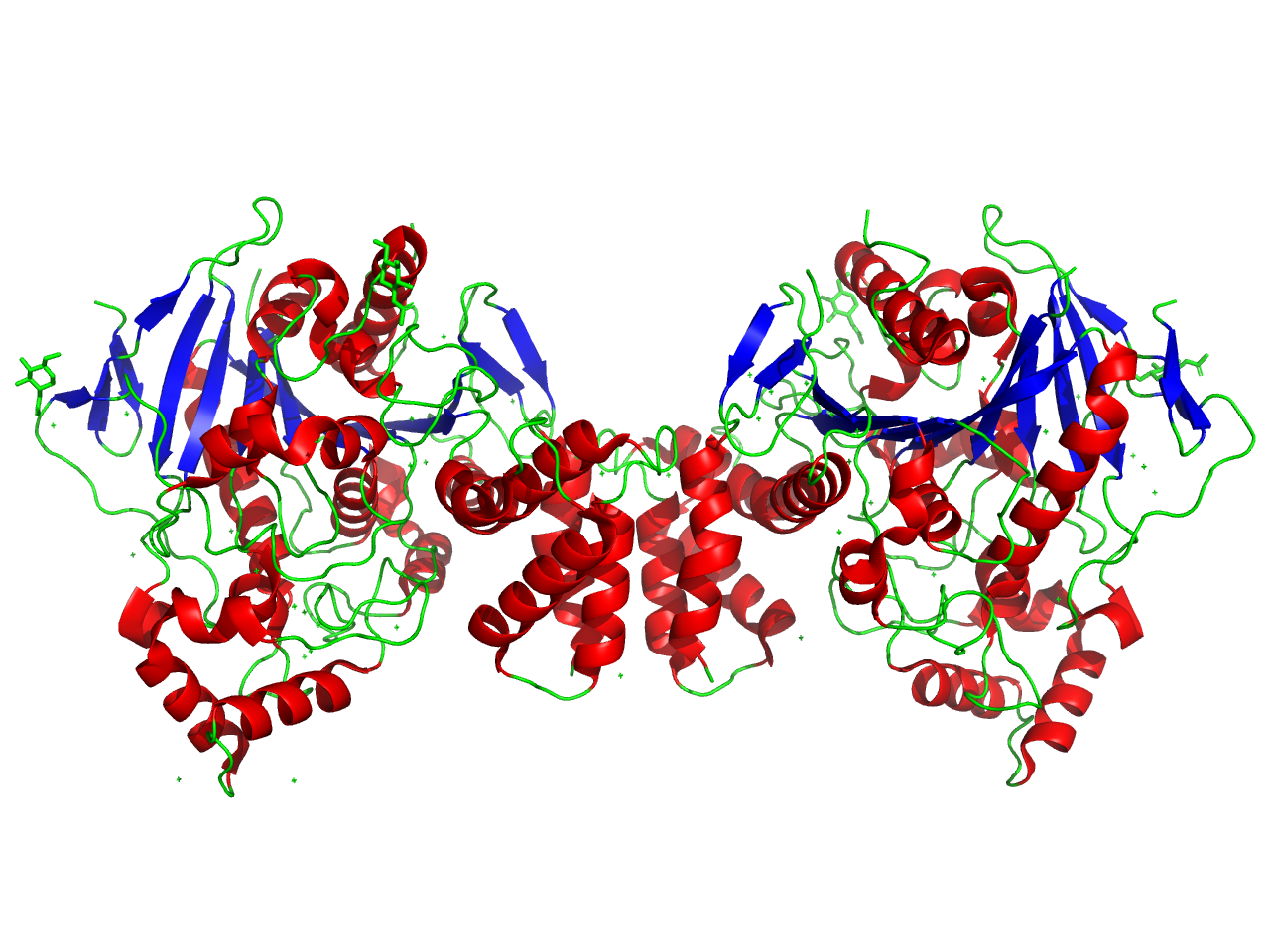
Above: Ribbon structure diagram of dimerized NLGN3. Image generated with PyMol by Ashleigh Waterman (Trinity ’26). PDBE code courtesy of Yoshida et al. (2021).
What is Neuroligin-3?
The brain is constructed of connections between cells known as synapses. For the brain’s communication network to function effectively, neurons must form precise connections with the right cellular partners in specific locations. This process, called synaptogenesis, is in part governed by cell adhesion molecules (CAMs)—proteins located on the cell surface that mediate binding between cells or with the extracellular matrix. In the brain, neurexins and neuroligins are two key CAM families essential for synaptic communication. Neurexins, located on the presynaptic cell, extend into the synaptic cleft and bind to neuroligins found on the postsynaptic cell, forming a trans-synaptic bridge. These bridges act as the brain’s “glue,” stabilizing synaptic connections and enabling efficient communication.
Neuroligins are a specialized type of CAM that play a vital role in maintaining synaptic function. Among them, Neuroligin-3 (NLGN3) is particularly interesting to researchers because of its versatility. While other neuroligins are selectively expressed in either excitatory or inhibitory synapses, NLGN3 is present in both. This dual role makes NLGN3 a crucial component in maintaining efficient neural communication. Researchers have linked disruptions in its structure or function to developmental and neurological conditions, including autism spectrum disorder (ASD). This article explores how NLGN3 works, why it’s important, and what its structure reveals about its role in brain development and function.
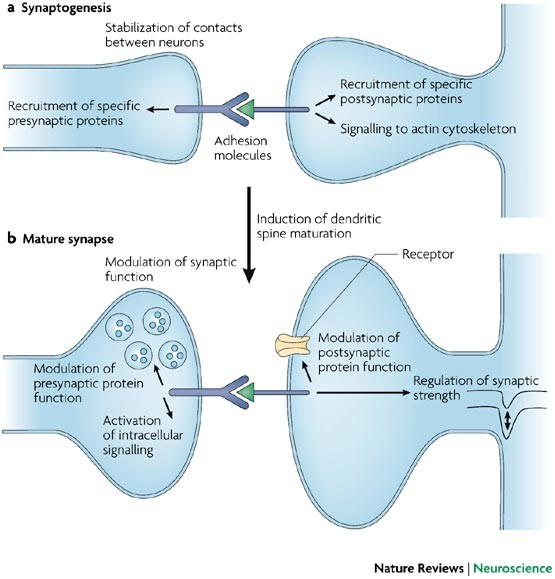
Above: Cell adhesion molecules stabilize synaptic connections between neurons. Image courtesy of Dalva et al. (2007).
Biological Mechanism
NLGN3 strengthens synapses in two key ways. First, it physically anchors the postsynaptic (receiving) cell to the presynaptic (transmitting) cell by binding to neurexins across the synaptic cleft. This connection forms stable complexes that link the two cell membranes. In the constantly changing environment of the synapse, even small shifts can disrupt alignment and communication. By stabilizing the structure, NLGN3 helps maintain the alignment of the cells, improving neurotransmitter signaling and maintaining the strength of the synapse.
In addition to physically linking neurons, NLGN3 helps organize molecules inside the postsynaptic cell to make signaling more efficient. It positions neurotransmitter receptors and scaffolding proteins like PSD-95 in their appropriate locations, ensuring the cell is ready to respond immediately to messages from the presynaptic cell. This organization allows signaling pathways to activate quickly, supporting processes like learning, memory, and adaptation. By stabilizing the connection between neurons and organizing postsynaptic components, NLGN3 keeps synaptic communication stable and efficient.
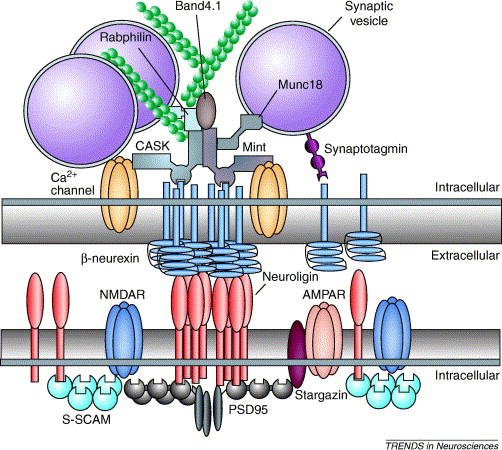
Above: Neuroligins (red) on the postsynaptic cell interact with neurexins (blue) on the presynaptic cell, linking the two cells together. Neuroligins also recruit intracellular signaling molecules for communication. Image courtesy of Dean et al. (2006).
Determining NLGN3’s Structure
To better understand how NLGN3 performs its function, a research group led by Dr. Tomoyuki Yushida from the University of Toyama used X-ray crystallography to model and study its structure. This process spans five key steps:
- Obtaining the Protein: Researchers extracted NLGN3 mRNA from a mouse forebrain, reverse transcribed it into complementary DNA (cDNA), and cloned this DNA into HEK293T cells. These cells produce large amounts of NLGN3 using their endogenous protein synthesis machinery.
- Purifying the Protein: To extract the protein, the cells were lysed to free all the cellular contents, including organelles, genetic material, and proteins. Using metal affinity chromatography, researchers isolated NLGN3 by binding it to beads coated with a specific antibody. Researchers washed, concentrated, and stored the isolated protein for later use.
- Crystallizing the Protein: Researchers used a method called sitting-drop vapor diffusion to form protein crystals. These crystals are essential for amplifying the diffraction patterns used in X-ray crystallography, which are the unique patterns of scattered X-rays that indicate the arrangement of atoms in a crystal.
- Collecting Data: Researchers collected X-ray diffraction data at a temperature of 100 K using the Spring-8 BL41XU beamline.
- Determining the Structure: Researchers used the molecular replacement method to determine NLGN3’s structure. Using the known structure of a similar protein, NLGN1, as a reference, they aligned NLGN3’s diffraction data to it to generate a preliminary electron density map. They refined the map to account for unique features of NLGN3, resulting in a detailed 3D model of its structure.
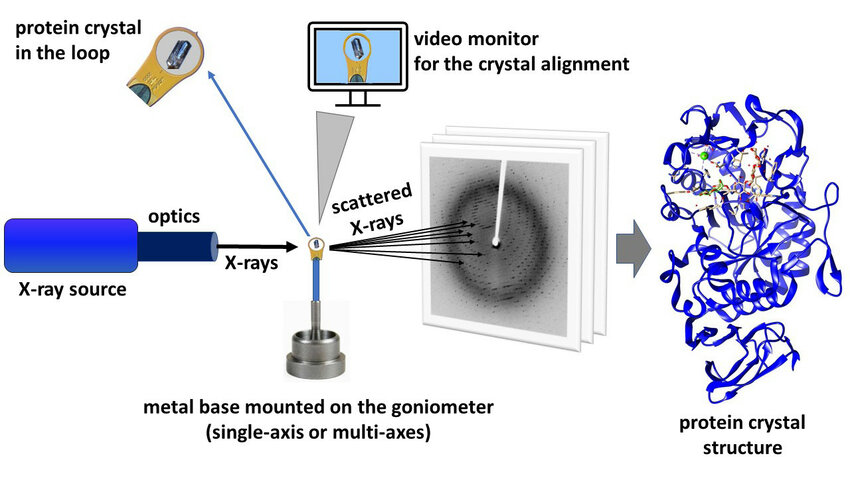
Above: Schematic illustrating X-ray crystallization process. Image courtesy of Kurpiewska et al. (2021).
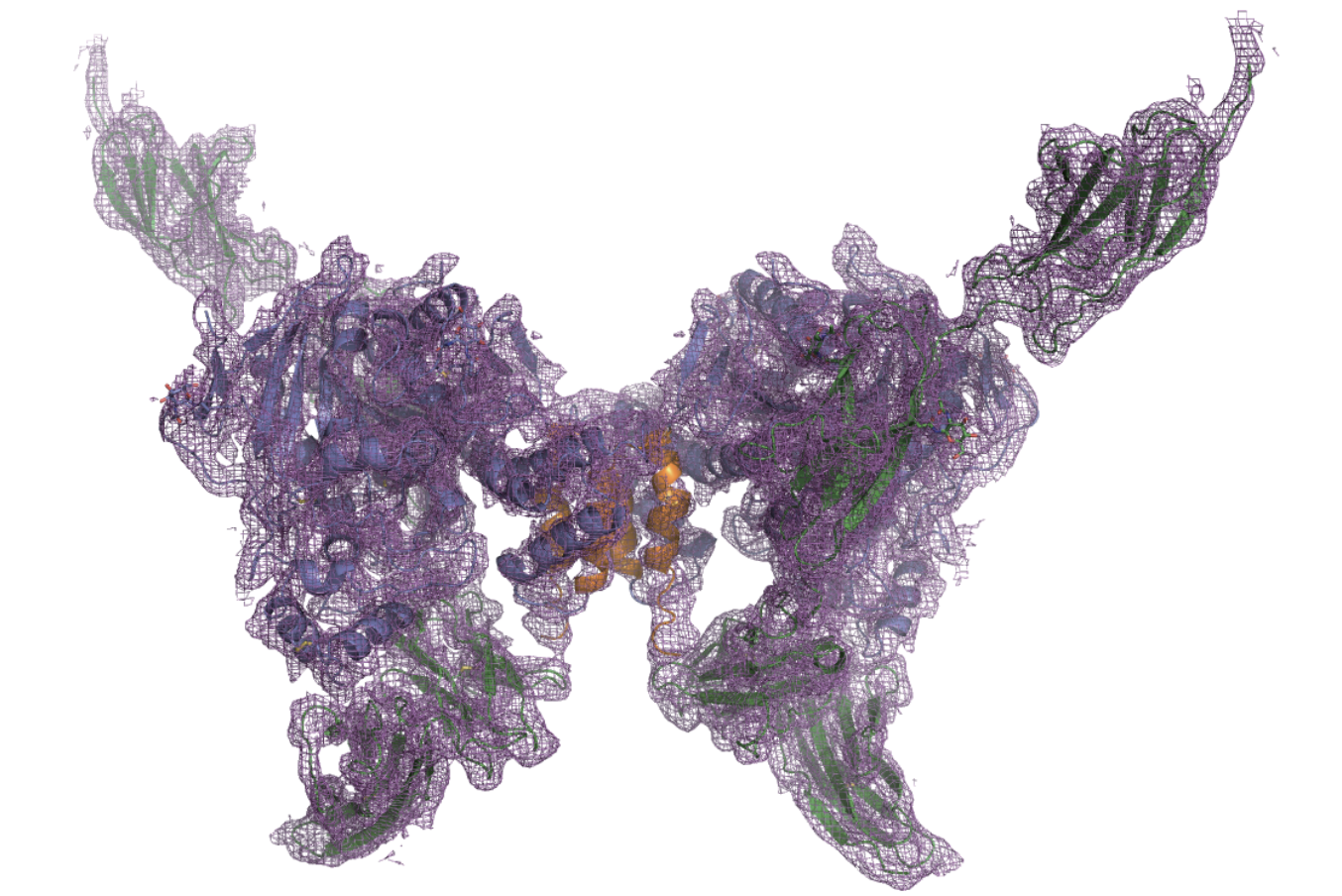
Above: Electron density map of NLGN3 produced via X-ray crystallography. Image courtesy of Yoshida et al. (2021).
Structural Overview of NLGN3
Like all proteins, NLGN3’s structure enables its function. NLGN3 is a single-pass transmembrane protein with three key components: a large extracellular domain, a single transmembrane helix, and a short intracellular domain. As part of the cell’s endomembrane system (a group of organelles that work to transport fats and proteins), NLGN3 is synthesized in the rough endoplasmic reticulum (RER), processed through the Golgi apparatus, and trafficked via the secretory pathway to its destination at the postsynaptic membrane. Each domain plays a distinct role in facilitating NLGN3's function in synaptic adhesion and communication.

Above: Overview of NLGN3’s key functional features, including glycosylation sites, transmembrane domain, and dimerization interface. Image generated with PyMol by Maya Chandar (Trinity ’26).
Extracellular Domain: The extracellular domain (ECD) is the largest and most functionally significant region of NLGN3. It features an α/β-hydrolase fold made of alternating α-helices and β-sheets which acts as a structural scaffold, enabling binding to presynaptic neurexins and supporting the formation of homo- and heterodimers. This structural role is crucial for NLGN3’s ability to stabilize and maintain synaptic connections.
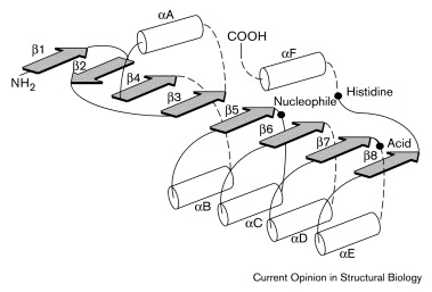
Above: Illustration of a typical α/β-hydrolase fold. Image courtesy of Nardini et al. (1999).
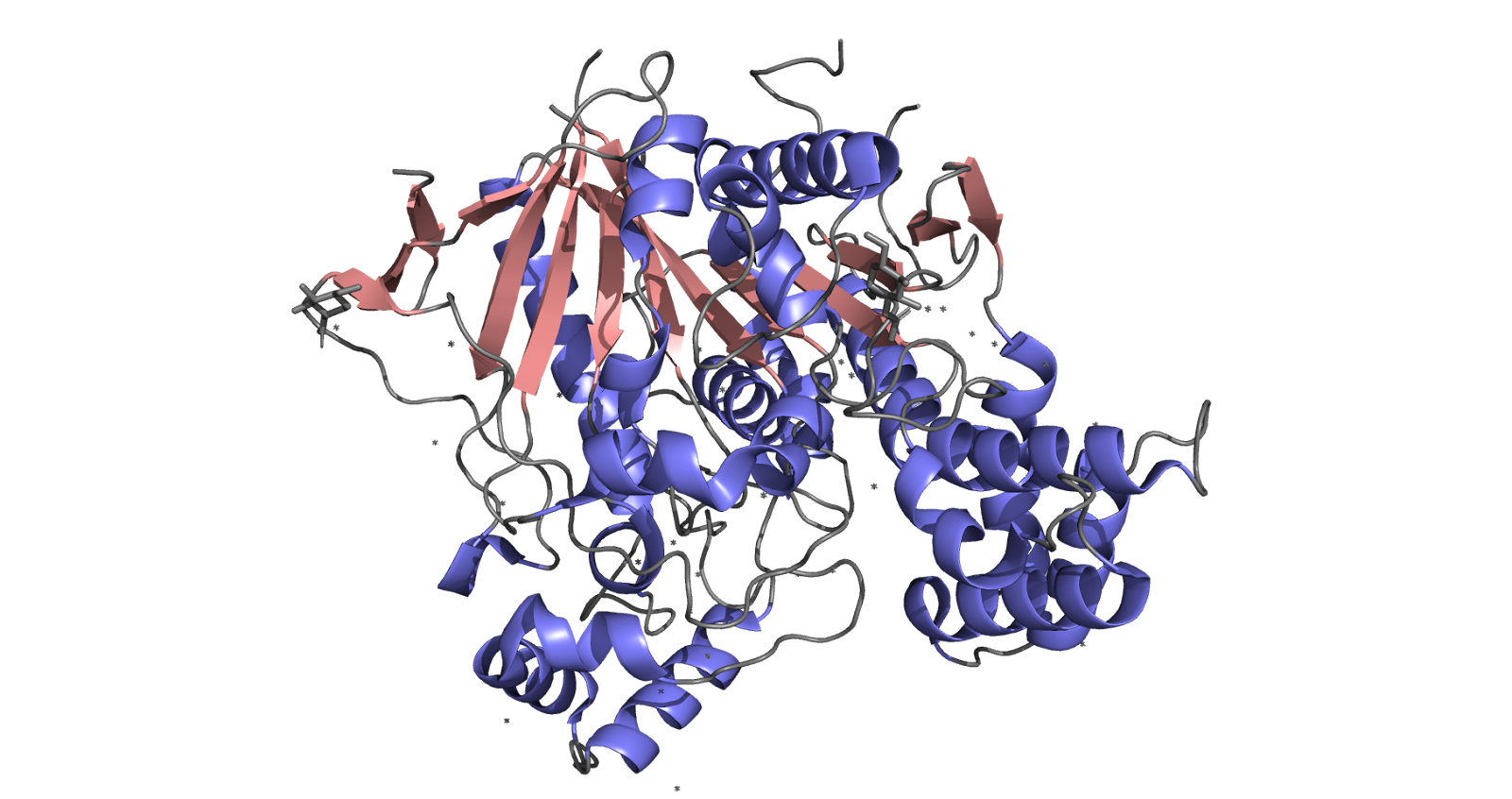
Above: Visualization of α/β-hydrolase fold in NLGN3 monomer (β sheets shown in pink and α helices shown in purple). Image generated with PyMol by Ashleigh Waterman (Trinity ‘26). PDBE code courtesy of Yoshida et al. (2021).
Transmembrane Domain: The transmembrane helix anchors NLGN3 within the postsynaptic membrane. Hydrophobic amino acids in this region interact with the lipid bilayer, ensuring stable membrane integration.
Intracellular Domain (ICD): The short ICD interacts with postsynaptic density proteins such as PSD-95, linking NLGN3 to the postsynaptic signaling machinery. These interactions organize downstream signaling pathways, enabling activity-dependent changes like gene regulation and synaptic plasticity.
Dimerization: NLGN3 forms homodimers or heterodimers, complexes consisting of two identical or similar molecules put together (e.g., with NLGN2 at hippocampal synapses) via its extracellular domain. This dimerization is essential for binding presynaptic neurexins, optimizing synaptic connectivity, and supporting plasticity.
Medical Relevance: The R451C Mutation and ASD
The R451C mutation in NLGN3 was the first genetic mutation linked to ASD. In biochemical terms, “R451C” refers to a substitution at position 451 in the protein sequence, where the amino acid arginine (R) is replaced by cysteine (C). Amino acids are the building blocks of proteins, and even a single substitution can significantly alter the structure and function of the entire protein.
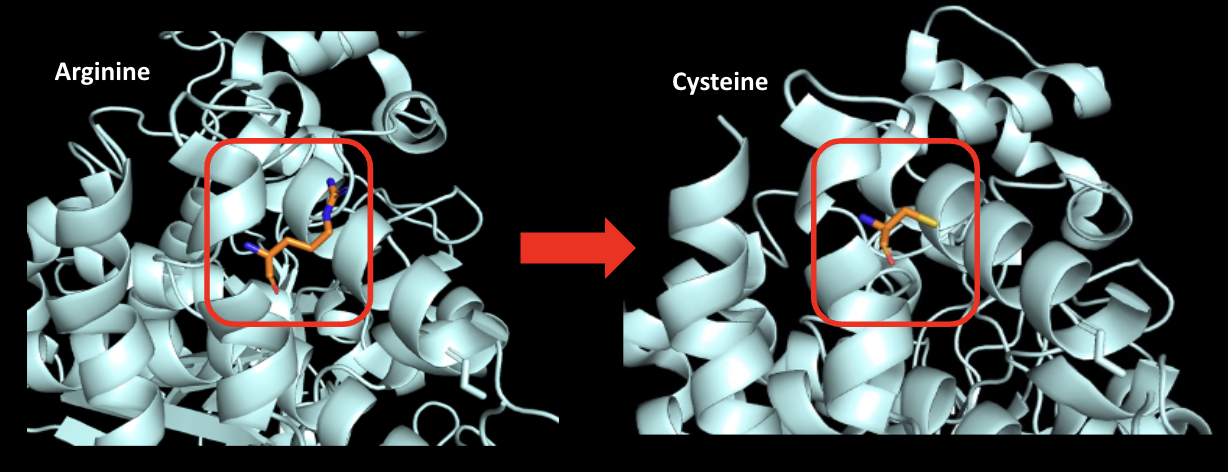
Above: Visualization of arginine mutation to cysteine at residue 451. Image generated with PyMol by Maya Chandar (Trinity ’26).
Several biochemical mechanisms may explain how the R451C mutation impacts synaptic function. Replacing the positively charged arginine with the uncharged cysteine reduces electrostatic interactions, weakening NLGN3’s affinity for binding partners. Cysteine’s lesser ability to form hydrogen bonds further disrupts interactions with other R groups, affecting the protein’s tertiary and quaternary structures. Unlike arginine, cysteine can engage in acid-base chemistry, potentially altering the protein’s reactivity and molecular interactions. Additionally, cysteine’s smaller size can lead to improper packing, compromising structural integrity. Another key factor is cysteine’s ability to form disulfide bonds, which may result in aberrant covalent interactions. Taken together, these structural disruptions trigger the unfolded protein response, leading to the degradation of NLGN3 in the rough endoplasmic reticulum (RER). The resulting loss of functional NLGN3 likely disrupts synaptic pruning, causing an excess of synapses. This surplus may contribute to experiences characteristic of ASD, such as sensory overload.
Implications
The detailed structure of NLGN3, as revealed through X-ray crystallography and captured in the 7CEE PDB structure, offers valuable insights into its role in synaptic adhesion and function. By modeling a significant portion of the protein, researchers have deepened their understanding of its mechanisms—particularly its interactions with neurexins and its role in synaptic plasticity. This knowledge is crucial for advancing our understanding of neurodevelopmental disorders and could inform future therapeutic strategies aimed at addressing disruptions in synaptic communication.