Solar-Powered Cells in Fuel? Scientists Engineer Yeast that Harness Light Energy
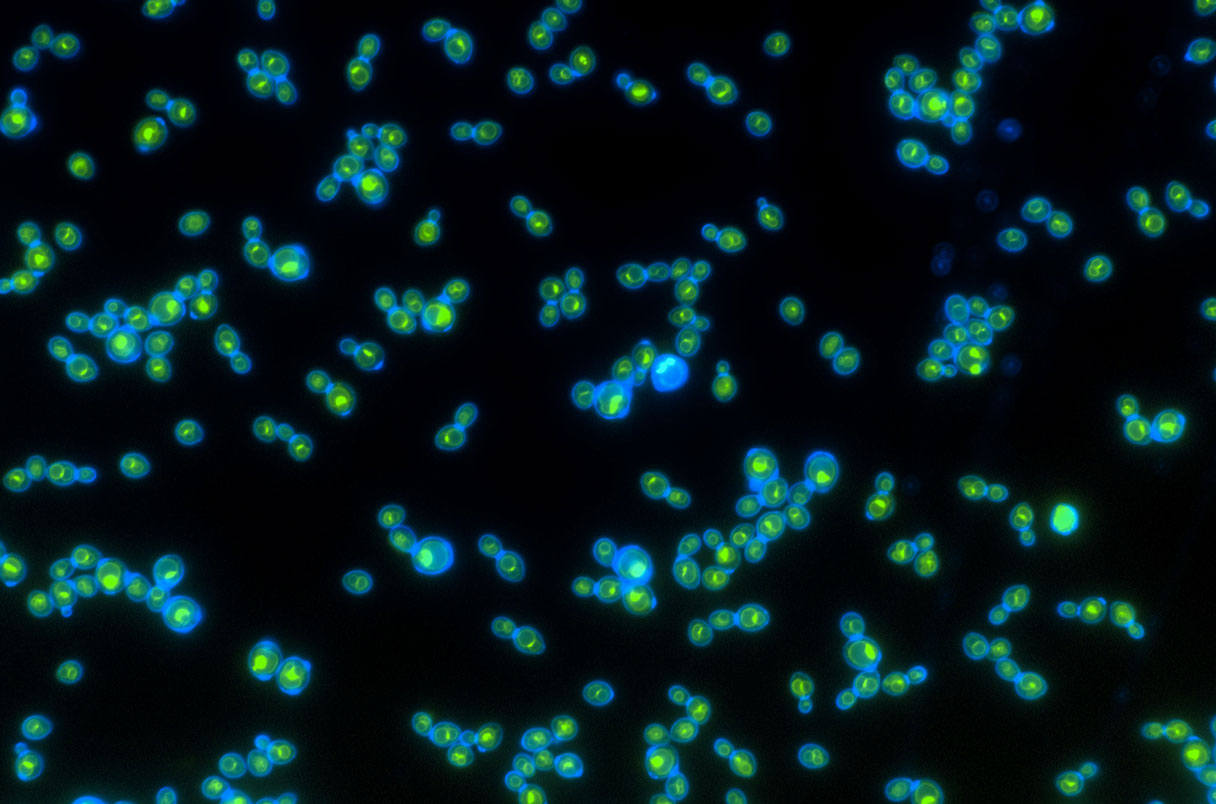
Above: Microscopic image of yeast cells with rhodopsin (in blue). Image courtesy of Science.
A new phototroph
Say it with me: photosynthesis. In first-grade science class, students around the world learn that plants are the only living things that can harness the power of the sun. However, scientists at Georgia Tech have recently proven this assumption wrong. The researchers team engineered yeast cells to actively mobilize energy from light, paving the way for future breakthroughs in regenerative science and alternative methods of energy production.
The evolutionary background of heterotrophs and autotrophs
To fully appreciate the significance of this scientific breakthrough, it’s important to first understand some evolutionary history. When the first cells emerged four billion years ago, they were, as one might expect, simple. These primitive prokaryotic cells were nothing more than a protective phospholipid bilayer plasma membrane (think of this as the skin of a cell), enclosing a semi-organized soup of organic molecules and RNA.
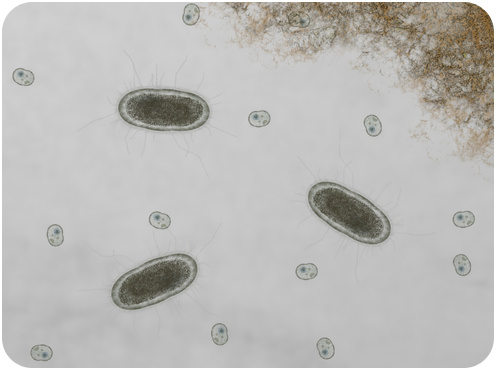
Above: Microscopic images of simple cells. Image courtesy of Biology Libretexts.
While gathering data on these early cells is quite difficult (as they did live billions of years ago), many scientists believe that these ancient cells were heterotrophs, which used biochemical pathways to obtain energy and extract vital organic compounds from an external source.
However, a world with only heterotrophs presents an existential threat to the continuity of life itself. As heterotrophs drained Earth’s finite resources, a new type of organism evolved: phototrophs. These organisms use light energy to drive metabolic processes, inventing the process of photosynthesis. While the first phototrophs were primitive cyanobacteria, complex eukaryotic phototrophs evolved through the absorption of these tiny bacteria by large heterotrophic host cells, as posited by endosymbiotic theory. Over millions of years, a clear distinction has evolved between heterotrophic cells and phototrophic bacteria.
Building solar-powered cells
The metabolism of photosynthetic organisms like plants and heterotrophs like yeast is fundamentally different. How did the researchers transform yeast, which has virtually no evolutionary history of phototrophy, into an organism that transforms light energy into chemical energy?
The researchers at Georgia Tech decided to solve this problem by engineering an alternative pathway to traditional photosynthesis. The mechanisms of photosynthesis within plants are extremely complex. Transferring the bulky machinery of electron transport chains, volatile oxidation reactions, and complicated carbon fixation into a single-celled organism like yeast would be a nearly impossible task.
Instead, these scientists decided to focus on a different photosynthetic pathway through microbial rhodopsins. Supporting the structure of rods and photoreceptors in your retina, rhodopsins are proteins that make vision possible. However, these proteins also have another significant function. Similar to the electron transport chain mechanism found in plants, rhodopsins can channel light energy into chemical energy by absorbing photons and using that energy to pump protons through an ATP synthase—without the involvement of any other organelles.
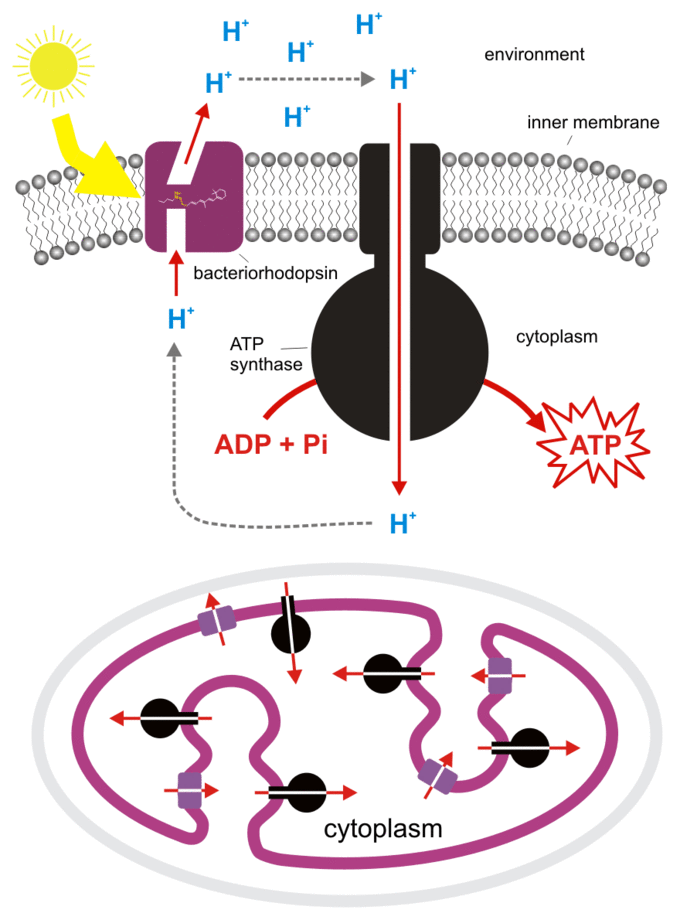
Above: Mechanism of ATP Production by rhodopsin proteins. Image courtesy of Biology Libre Texts.
While studying microbes found at the ocean’s surface, several scientists from USC found that rhodopsin-driven photosynthesis can absorb just as much light energy as traditional chlorophyll-based photosynthesis. This finding suggests that rhodopsins could be a viable tool for engineering photosynthetic systems. Building on these results, another group of scientists from Oxford managed to successfully create an artificial photosynthesis system using rhodopsins in place of chlorophyll.
The simplicity and viability of rhodopsins as a light-absorbing pigment captured the attention of Georgia Tech researchers. To test the application of such a protein in a living organism, researchers extracted a rhodopsin gene from a parasitic fungus, which expresses the rhodopsin protein in a cell’s vacuole.
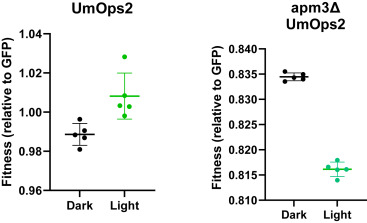
Above: Comparison of the fitness of rhodopsin yeast (encoded by the rhodopsin gene UmOps2) growing in green light versus growing in the dark. Image courtesy of ScienceDirect.
After inserting the gene into yeast cells, the researchers observed that, when illuminated, the yeast with the rhodopsin gene grew up to 0.8% faster than yeast without the gene. Rhodopsin yeast also grew up to 2% faster in the light than in the dark.

Above: Comparison of the number of dead cells between rhodopsin and non-rhodopsin cells in different conditions. Image courtesy of ScienceDirect.
A caveat in this photosynthetic model is that yeast with rhodopsin had a much higher mortality rate, suggesting that the rhodopsin gene induced a high reproduction rate to overcome a high mortality rate. Researchers surmised that this may be due to the proton-pumping effect of rhodopsin on the vacuole. While this function destabilizes the pH of the cell, causing protein denaturation and rapid death, the increase in overall energy allows greater reproduction rates.
Ultimately, their study showed that the yeast successfully incorporated rhodopsin into its metabolic system, becoming a hybrid photoheterotroph.
What a photosynthetic Saccharomyces cerevisiae could do
While a 2% higher growth rate seems fairly insignificant, the findings from this study have massive implications for countless industries.
In terms of evolutionary biology, the success of transplanting a parasitic rhodopsin gene into a yeast cell may shed light on ancient mechanisms of evolution. By creating a small-scale model of horizontal gene transfer, a process by which distantly related organisms can exchange genes, scientists may have demonstrated a way that the rhodopsin gene spread across vast lineages.
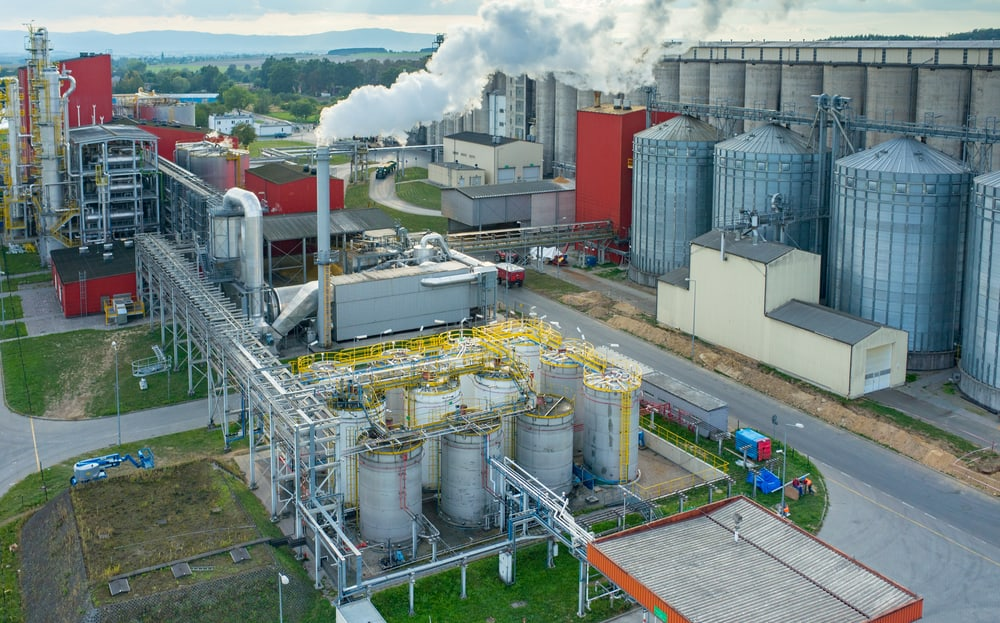
Above: A biofuel production factory. Image courtesy of Freight Waves.
Beyond the lab, photosynthetic yeast could spark new advancements in fields ranging from biofuel production to agriculture. Creating photoheterotrophic organisms may diversify and enhance the production and potential of biofuels. Unlike hardy plant cells, which have tough cellulose cell walls, yeast cells are much easier to break down in the process of producing biofuels, demonstrating the value of yeast in making biofuels a viable energy source.
Lastly, the results of this experiment could even lead to new developments in regenerative science. Known as the lysosome of the yeast, the yeast cells’ vacuoles play an important role in cellular aging and autophagy. Since rhodopsin is directly inserted into the yeast’s vacuole, future investigations of rhodopsins in yeast cells may lead to new discoveries in slowing down cellular aging.
By transporting a singular gene across organisms, researchers succeeded in opening a gateway to vast, new innovations in many fields, showing just how impactful genetic engineering can be for our world.