Synapse Shutdown: Tracing Neuronal Inhibition with Phosphorylated Pyruvate Dehydrogenase
Donald Hebb famously said that “cells that fire together wire together.” This mantra has guided neuroscientists for decades, and much of contemporary neuroscience and neurobiology research has focused on action potentials and activity-dependent connections in the brain. Scientists have employed techniques such as electrophysiology, calcium dyes, and the use of intermediate early genes (IEGs) to study these intercellular signals and firing patterns. Although each technique has its advantages, tracking the expression of intermediate early genes can provide neuroscientists with a target for staining, allowing them to trace and visualize excitatory pathways under a microscope using fluorophores.

Above: Visualization of information traveling between neurons. Image courtesy of the Zenke Lab.
While tracking neural activity is one crucial aspect of neuroscience research, scientists have yet to develop robust techniques to track and isolate inhibited neurons for projection studies. Historically, scientists have depended on recordings and calcium-based methods to study neural inhibition. To study activated pathways during a specific assay or behavior, many neuroscientists have stained for Fos, a protein whose expression is strongly correlated to neuronal activity. But until now, staining for an equivalent to Fos for inactivated neurons has not been an option.
“For decades, scientists have studied the intricate activity patterns in human and animal brains by observing when different groups of brain cells turn on. Equally important to understanding the brain and related diseases, however, is knowing how long those neurons stay active and when they turn off again,” the Scripps Research Institute wrote. The ability to study these patterns of activity and inactivity is especially useful to unravel neurological mechanisms behind PTSD, depression, and Alzheimer’s, among other disorders. A research team at Scripps led by Drs. Le Ye and John Yates recently shared new findings on their simple approach to tracking inhibitory responses to stimuli using an inverse activity marker.
The California-based research team found that during inhibition, levels of non-phosphorylated pyruvate dehydrogenase (PDH) decrease at rates much higher than any changes observed at the genetic level. Previous work on PDH has revealed its critical role in metabolism and energy production, but this research reveals a novel use for this enzyme in projection studies.
Why did Ye, Yates, and colleagues target this specific protein? The team conducted a phosphoproteomic screen, or an objective search for cellular events correlated with neuronal activity. They first synchronized firing patterns in cultured neurons using an optogenetic technique to produce action potentials after exposure to light. When the neurons fired, the team detected the expression of well-known IEGs that mark neuronal activity. They then searched for proteins whose phosphorylation states change at low neuronal activities. The group identified multiple phosphorylation sites on a PDH subunit that showed promise as a target for this research, particularly because monoclonal antibodies for phosphorylated PDH (pPDH) are already highly refined and widely available.
Biochemical and behavioral assays told the rest of the story. Harnessing the knowledge that anesthesia induces neural inhibition, researchers found high levels of pPHD expression in anesthetized mice, indicating a role for this enzyme in inhibitory patterns. In further experiments, the researchers exposed mice to complete darkness followed by bright light for several hours and a secondary period of darkness. They observed that while activated neurons labeled with FOS took 12 hours to fully subside following the period of light exposure, levels of pPDH in the visual cortex, lateral geniculate nucleus, and lateral entorhinal cortex increased only two hours following reintroduction to the dark environment. These findings suggest that pPDH staining provides rapid visualization of inhibition in the visual pathways.
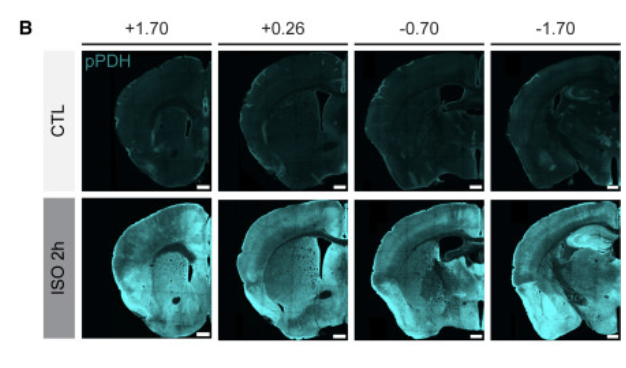
Above: pPDH labeling in the brain of control mice versus mice anesthetized with isoflurane for two hours. Photo courtesy of Ye et al.
pPDH tracking is an exciting new technique for disease research. In addition to disorders like Alzheimer’s and PTSD, the paper highlights possible applications to the study of metabolism, hunger, appetite, and obesity. For example, in one assay, the group used pPDH staining and fasting-refeeding experiments to characterize activity and inhibition patterns in a specific population of neurons in the lateral hypothalamus.
When asked about the future of this new approach, Dr. Ye said, “There are a lot of questions that this technology can help us answer. If the brain can’t turn cells off, or if they’re turned off faster or slower than usual, what happens? How does the inhibition of neurons play a role in different diseases?” With the breakthrough of pPDH-specific labeling, scientists can now tackle these exciting questions.